Podcast: Play in new window | Download
Subscribe: RSS
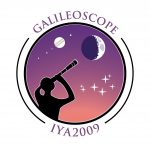
Galileoscope Logo
How can you get a Galileoscope of your very own? What happens to time inside a black hole? And what exactly is energy anyway?
If you’ve got a question for the Astronomy Cast team, please email it in to info@astronomycast.com and we’ll try to tackle it for a future show. Please include your location and a way to pronounce your name.
|
Shownotes
How can I find a Galileoscope?
Does time stop inside a black hole?
- Time in a black hole — Ask an Astrophysicist website
- Does time slow down near the event horizon of a black hole? — Ask an Astrophysicist
- The Anatomy of a Black Hole –UIUC
- Interactive Anatomy of a Black Hole — Think Technologies
- Nancy Atkinson (hi everyone!)
What exactly is energy?
- Energy – Wiki
- The different forms of energy — NMSEA
Can energy be converted back to matter?
- Can energy be converted to matter — Newton Ask a Scientist
- Large Hadron Collider
- The End of Everything –– Universe Today
- Episode #87: End of the Universe, the End of Everything
Why don’t we put space telescopes like the Hubble or JWST on the International Space Station so that it would be easier for astronuats to service them?
- Vibration Isolation systems for the ISS
- Google book: Guidelines for Noise and Vibration levels for the Space Station — National Research Council
- ISS
- Hubble Servicing Missions
- James Webb Space Telescope
- WMAP
Is there a limit to how far in space we can see?
- Cosmic Microwave Background — U of Chicago
- Can we look far enough back in space and time to see the Big Bang? — Cornell U
Why can’t the most powerful photons like gamma rays and X-rays make it through our atmosphere, but on Earth we need lead to protect ourselves from them, while less powerful photons like light come through the atmosphere but can’t make it through a piece of paper?
- Gamma rays coming through the atmosphere — Ask an Astrophysicist
- Solar EM Radiation in Earth’s atmosphere (about 1/3 of the way down the page) — Windows to the Universe
- Ep. 132 — Infrared Astronomy
- Ep. 133 — Optical Astronomy
- Ep. 134 — Ultraviolet Astronomy
- Ep. 135 –X-ray Astronomy
- Ep. 136 — Gammy Ray Astronomy
We’ve heard about the tidal heating of Europa, Enceladus and Io, but how much of Earth’s internal heating is due to lunar and solar tidal forces, and how much is due to radiation energy and heat left over from the Earth’s formation?
- What heats the Earth’s core? –Penn State
- Radon — Wiki
- Tidal Force — Hayden Planetarium and Neil de Grasse Tyson
Questions Show: Galileoscope, Black Hole Time, and What Exactly is Energy?
Fraser Cain: Welcome to the AstronomyCast questions show where we answer your questions about space and astronomy. If you have a question for the AstronomyCast team please email it in to info@astronomycast.com and we’ll try to tackle it for a future show. Please include your location and a way to pronounce your name.
Hi Pamela, more and more questions. [Laughter]
Dr. Pamela Gay: They just won’t stop coming.
Fraser: Oh and I just wanted to give you a quick prop. There was a recent review of astronomycast on iTunes which was wonderful. Thank you everybody who has been sending them.
We may have like 600 and something. Someone said sometimes the shows seem a little scripted. I just want to make it clear – there’s no script.
Pamela: Yeah.
Fraser: We’re too lazy for scripts. That would be work. In many cases you have no idea what I’m going to ask you.
Pamela: No. I have absolutely no idea.
Fraser: In some cases we fix when we go down in some wild goose chase in editing and maybe we’ll re-record. But most of the time it’s one cut. I’m asking whatever I want and you are answering them from your vast repository of knowledge which I think is amazing. There’s no script.
It’s time to test you one more time. How can you get a Galileoscope of your very own? What happens to time inside a black hole? What exactly is energy anyway?
The first question comes from Chris Lingre “Fraser and Pamela mentioned a $15.00 telescope in a question show. What are they talking about? I haven’t been able to find one and had no luck locating one online.”
What are we talking about? We’re talking about the Galileoscope.
Pamela: Yes. This is a wonderful project that comes out of the International Year of Astronomy. A group of entirely volunteer folks sat down and designed an amazing telescope that is being sold for about $15.00 plus shipping through galileoscope.org.
We’ll have a link in my twitter feed and the astronomycast twitter feed and as well as on our website. This telescope comes with a Barlow lens, two different eyepiece lenses. You can do all of the observations that Galileoscope did with it and you can do better than Galileoscope.
What’s really cool about it is with one of the eyepiece combinations you’re looking through a system absolutely identical to Galileo’s. Everything is upright. It’s really bad eye relieve, really tiny field of view. It’s just miserable and gives you a real appreciation for what Galileo was able to accomplish.
Take that out, replace the eyepiece and all of a sudden you’re seeing through this really nice 50X telescope. What’s cool is we’ve had Alan Nagler look through it. He actually put one of his really nice eyepieces on it and he was amazed by the view that he saw.
Some really good folks including Rick Fienberg, Editor Emeritus of Sky and Telescope, Steve Pompea of the National Optical Astronomy Observatory, Doug Erin up in Wisconsin, a whole team of people worked together, tested this.
Not only is it a really good telescope but if you’re a school teacher you can also take the telescope apart and use it as an optical bench. You have an optical kit. You have a diverse telescope that mounts on a standard tripod. It’s just a really nice system. Like I said, it’s $15.00 plus shipping.
Fraser: And we’ve both tried them out. I tried it out at the last AAS in winter in California and it was great. It was just great. We looked at Jupiter and we could see the moons of Jupiter. We could see bands on the planet.
You can see the rings of Saturn. You can see beautiful craters on the moon. We looked at the Orion nebula. This is the real deal, it’s a $15.00 telescope, and it works.
Pamela: You can use it with a webcam. It’s really lightweight so if you’re trying to mount a heavy camera on it, not so good. For a standard webcam for a lightweight digital camera it works excellent.
They’re awesome. Buy them for your entire school. That’s the cool thing about this. There are a lot of communities that have gotten together as a community and bought a telescope kit for every single kid in say the 6th grade, in the 8th grade when they’re still young enough to be interested and enthusiastic.
At $15.00 each you can host a local star party for a local classroom of kids and get the local supermarket to sponsor sending the kids home with the telescope they used at the star party.
Fraser: Definitely check it out at www.galileoscope.org and order one, ten, the more people can order then that can help bring the cost down for the volume and make them really stick around.
I think this is going to be a great way to just get more and more people involved and interested in astronomy. It’s the International Year of Astronomy. This is a great way to get involved.
Pamela: Just like with the one laptop per child, you can buy a scope for yourself and buy a scope for a kid in a third world nation that otherwise would never get to use a telescope. Buy one for yourself and buy one for someone you don’t even know and give them a chance to look up and see the stars.
Fraser: Awesome. We have our second question from Nancy Atkinson. If anybody doesn’t know who Aunt Nancy is, she’s a senior editor over at Universe Today and does a lot of the writing on the Universe Today. She actually does the show notes for Astronomycast and is the producer for 365 Days of Astronomy. She’s a part of the team.
Pamela: She keeps us sane. [Laughter] We couldn’t do this without her.
Fraser: Oh yeah. But her other job is a librarian and she got this question at the library. “Does time stop in a black hole?” Which I guess is a good question.
Pamela: It’s a really good question.
Fraser: Why would it stop? Why is it even possible?
Pamela: In the limit that velocity goes to the speed of light the speed of time goes to zero. This is a kind of squirrelly way of saying that the passage of time for someone who is traveling at the speed of light – which you can’t ever do – but if you were able to go at the speed of light – which you can’t do – then your watch would stop ticking.
Your perception of time as neurons in your brain fire would stop as well because well everything would stop for you. Since things can’t actually go the speed of light as long as they’re regular matter time will still exist.
The thing is we don’t know what happens to matter when it becomes part of the black hole. There is stuff falling in – it’s still normal matter. It’s not traveling at the speed of light. It’s just stuck inside the event horizon. Once you start to get to the physical stuff that makes up the black hole, it’s not regular matter in any way that we understand.
We don’t know how to understand it. We know that with normal matter you have regular stars. You collapse it down and it becomes degenerate gas and you get white dwarf stars. Collapse it down more and you get neutron stars.
Collapse it down more and we don’t know what it is. It’s a black hole and it’s made of stuff. We don’t know what to call that stuff because quantum mechanics and gravity don’t speak nice to each other so we don’t know what to do.
As long as the stuff is stuff with mass it’s not traveling the speed of light. It still has some perception of time. It’s probably really slow because we’re dealing with gravitational red shifts.
We’re dealing with it is moving fast before it goes splat. If it becomes pure energy somehow, energy is light and light travels at the speed of light. Light does not experience time so if stuff that becomes part of a black hole becomes pure massless energy then time can stop.
Fraser: I guess part of it though is we don’t even understand does the contraction inside of a black hole ever stop, right? One idea is that the black hole is just some super exotic matter that just sits there and more gets munched on top of it.
It’s very small and very dense but we don’t really know what it is. Another theory is that it’s a singularity that it never stops collapsing.
Pamela: Right and we don’t know.
Fraser: We just don’t know. Good, we don’t know. Moving on, Robert Elms from Brisbane, Australia asked: “Can you please spend a few minutes to explain what exactly energy is?”
The universe is filled will all types energy including matter which we mention is frozen energy. Okay so let’s just deal with that. What is energy?
Pamela: That is one of the hardest questions. We were actually back the first year we were recording astronomycast on a morning radio show in Australia and they asked us this question and we stumbled over it for a while.
At the end of the day the best definition I have for what energy is would be it is something that can do work. It is something that can change direction. It is something that can change velocity. It is something that causes things to happen.
That’s rather unsatisfying but that’s really at the most general level. Energy creates light, causes vibrations, increases velocity and changes the direction of velocity. Energy does all those things.
Fraser: We can have potential energy which is just a rock sitting on the top of a hill, waiting to do work. It has energy in the system. If you let it roll down the hill and if you ignore friction, the waiting energy will turn into moving energy.
Then when it crunches into something it will turn into other kinds of energy. So, it is the ability to do work. I think then Robert is trying to ask “But what is it really?”
Pamela: [Laughter] that’s a question for a philosopher. At some point we need to store up all these questions and find a philosopher and hold them hostage.
Fraser: Naw, a philosopher would be no good to us. But so a photon is energy, a rock sitting at the top of a hill has energy. An elastic band stretched out has energy.
Pamela: The gas in the room that each of us is in – the gas that we’re breathing – it has energy.
Fraser: Yeah, now Robert has sort of a second question which is: “Can energy be converted back to matter?”
Pamela: Yes.
Fraser: It can?
Pamela: Yes. This is actually what happens in particle accelerators. You take two protons or two particles in general – you can do this with all sorts of different particles depending on which accelerator you use – but in general you can take two protons and get them in circles really fast in opposite directions.
One is going clockwise the other is going counterclockwise doing parallel donuts, going really, really fast. Then using magnetic fields you change their paths so they collide exactly head-on.
That’s what is really amazing is they can take two protons, accelerate them to a significant fraction of the speed of light and then get them to hit head on into one another. That’s just cool.
In the process of accelerating we’ve given them kinetic energy. The faster they go the more kinetic energy they have. Kinetic energy is defined in the classical sense as one half the mass times the velocity squared.
When they collide they come to a sudden grinding halt. In the collision all the energy that was in their kinetic energy as well as the energy that was tied up in their mass gets changed into all sorts of crazy stuff including in some cases we hope, the Higgs boson. This is what the Large Hadron Collider is hoping.
Fraser: But you get a shower of particles that are frozen energy. The speed of the particle – the velocity – gets turned into new particles.
Pamela: Yes, new particles that flash out in all different directions. We catch them in webs of fiber optics and we measure the velocity, their passage through the fibers. We measure their mass. We measure as many different things as we can.
Then we backtrack and put the pieces together and go aha, there was one particle here. That one particle decayed into these two particles and light. We’re able to reconstruct how the matter and energy flashed back and forth between having mass and being light and being both mass and light throughout the process of the collision and the shower of particles and the decays that follow after.
Fraser: Right now with our current technology all you can do is smash protons together and get a shower of particles. It is theoretically possible that you could smash particles together out could come trucks and puppy dogs and water and sandwiches.
Pamela: Right. At a certain level you and I are converting mass into energy every moment. We’re just not doing it via nuclear processes. We’re doing it via chemical processes.
Fraser: That’s the other way, right? That’s the opposite. We’re talking about how we’re getting energy turned into matter.
Pamela: Right that’s true.
Fraser: Then obviously we can turn matter into energy using a chemical process or just take antimatter and matter – put them together.
Pamela: That’s a bit violent but it works.
Fraser: Don’t you get two pieces of matter turning purely back into energy? [Laughter]
Pamela: Right you get two bits of mass – one matter and one antimatter – that if you have the right channel so electron and positron work really well at this. You collide them together and you get pure energy.
Fraser: Or a sandwich and an anti-sandwich. [Laughter]
Pamela: Exactly.
Fraser: Those will turn into pure energy. We can go from energy to matter and we can go from matter to energy and in a nuclear explosion, right? That’s matter to energy.
Pamela: Right.
Fraser: I guess Robert’s last question: “Will energy that exists today turn into matter or will matter turn into energy, or are we just kind of stuck with what we’ve got?”
Pamela: It’s a little bit of both. We use energy in all sorts of different processes. Some of the stuff that exists as energy today – that’s getting generated and a star getting generated, nuclear processes – some of that energy is going to freeze out into different particles eventually.
At the same time when you look really long term, when you look at the inevitable death of the universe – which has to come up once per episode – one of the things that a lot of particle theorists think may be true is that protons will decay.
This means that we’re looking at an eventual future where all matter decays. We’re left with basically a fuzz of energy spread out across this extremely, extremely large cold, cold universe.
Fraser: Right and so that might be the future that all the matter will just turn into energy and then it all gets spread out and that’s that. Energy – what is it? [Laughter]
Let’s move on. Mitchell Shyat from Oakland, CA asked: “Why can’t we just attach telescopes like Hubble or James Webb to the International Space Station or at least placed in an orbit nearby and that way if something goes wrong it could be fixed immediately?”
So, why didn’t they attach Hubble to the International Space Station somehow? The astronauts could just stick their eyes up right up to the eyepiece of the Hubble Space Telescope and take a look. [Laughter]
Pamela: People move – that’s the real problem. Because people do things like bump into the sides of the International Space Station and tap their fingers on the side of the station, the station constantly is vibrating.
The station has fans to control the air processing system. It has fans to control the waste processing system. It has all these different things that generate all sorts of different vibrations. All those vibrations would wreck images. You don’t want to attach telescopes to anything that human beings are attached to.
In fact when we build big observatories here on the planet Earth they’ll actually build the telescope on its own pier that isn’t attached to the building. There is usually an air gap of several inches all the way down through the center of these big observatory buildings between the floors that people work on and the actual pier of the telescope which goes all the way down to bedrock.
You’ll have this separate foundation for your telescope and for the building built around that telescope just to isolate the vibrations.
Fraser: You’re going to have heat coming off the space station which is going to cause problems. There’s going to be light pollution perhaps coming off the station.
Pamela: And then there’s just the well you have to contend with astronauts. You have to contend with the space shuttle.
You have to contend with the different supply vessels. The International Space Station is pretty complicated. Let’s not add a telescope.
Fraser: They sort of split the difference with Hubble. They put it in Earth’s orbit and made it so that it could be picked up and serviced by the shuttle. I think with James Webb they just said what’s the best place to put a telescope? Let’s put it there and not think about how we’re going to repair it.
This is what was done with WMAP. It is out in the LaGrange Point. A nice stable, dark place free of electromagnetic radiation and heat and all that. That’s just a good place for a telescope.
I guess that’s it, right? Some places are good for telescopes and some places are good for you to go and work on them. In the case of those telescopes they’re going with best place for telescopes – science first.
Pamela: Exactly.
Fraser: Daniel Ebdriptasked: “In episode 136 Fraser said that there is no minimum to the wavelength of the radio spectrum but isn’t the Planck length a theoretical minimum of anything including radio waves?”
I had said that I guess no minimum wavelength. In other words you could go past gamma rays and get smaller and smaller wavelengths.
Pamela: Which we’d still call gamma rays.
Fraser: Which you’d still call gamma rays even if they were 100 times more powerful, 1000 times more powerful, a billion times more powerful. Those would still be gamma rays.
You could keep on going forever shortening the wavelength and still be well within the laws physics. Daniel says: “Whoa not so fast. Isn’t the Planck length the limit?”
So what’s the Planck length?
Pamela: The Planck length is length scale at which gravity should be quantized. This is when we start trying to figure out if I look for natural units, if I start figuring out at what point does everything get quantized? At what point does everything start behaving following quantum mechanics?
I end up with certain Planck masses, Planck times and a Planck length. In theory you can’t ever get smaller than any of these things without entering pure quantum mechanic chaos. Everything is determined by probabilities slant.
The Planck wavelength is four times ten to the minus 35th of a meter. It’s really, really tiny. He’s right, he caught us. We goofed. You in theory shouldn’t be able to have a wavelength that is smaller than this ten to the negative 35th of a meter.
Kudos to him. If you do go the other direction, if you start looking at longer and longer wavelengths, there I don’t think we have a limit other than the size of the universe.
Fraser: Right so a universe length. Ramez Nadum from London asked: “Is there a limit to how far into space we can see even if we built the most powerful telescope possible?”
Is there a limit?
Pamela: Yes.
Fraser: There is a limit. What is that limit?
Pamela: The cosmic microwave background. It is this kind of impervious wall behind which we can’t see. It’s where the universe becomes opaque. Prior to the cosmic microwave background radiation decoupling from matter the entire universe was as opaque as the densest fog that you’ve ever been in with your high beams turned on.
Light tried to move through space but it was constantly getting absorbed and readmitted by the extremely dense material around it. So you have this complete coupling of light and matter and we can’t see through that opaque dense period in the universe. We can only see the moment at which they decoupled and the cosmic microwave background is released.
Fraser: So we already have instruments that can see this limit.
Pamela: What we’re working to build now is instruments to allow us to see really well the stuff between us and that limit.
Fraser: Right and that’s where we can only see galaxies with any resolution out to a few billion light years away and use special tricks. The James Webb telescope is going to come along and extend that view much, much further out.
What would you say right now is sort of a limit for what we can see with our best telescopes? Like with Hubble doing its deep field view?
Pamela: We’re able to see things from a few million years after the cosmic microwave background. We’re limited to only being able to see the very brightest, very largest objects that are in the process of forming.
It’s the Holy Grail is being able to start seeing the normal objects at the beginning of the universe. To start seeing regular galaxies in their infinite stages as they’re just starting to collapse out of many different combining small blobs of gas.
Fraser: Right to try and get a sense of what those first stars were.
Pamela: Exactly. We’re struggling right now to be able to see the normal stuff at the beginning of the universe as well as the big giant really glowing bright stuff.
Fraser: Okay. John from Milbrook, Ontario asked: “Why is it that the most powerful photons, gamma rays and x-rays, can’t make it to the surface of the Earth through our atmosphere but they require lead to protect us on the ground? Why can the less powerful make it through the atmosphere but can’t get through anything thicker than a piece of paper, like light?”
That’s a good question. Gamma rays will blast your DNA out if you let it and yet it can’t make it through the atmosphere. Light can make it right through the whole atmosphere but can’t go through a piece of paper.
Pamela: The beauty of this is the depths of which we expect things to travel through. Our atmosphere is conveniently thick. This is one of those really nice things that allow airplanes to be able to fly several miles up.
The higher up you go on a mountain the more radiation you’re actually going to start encountering, the more UV you’re going to be able to pick up. We can actually fly balloons high enough still within the atmosphere that they’re starting to pick up microwaves that are otherwise blocked by our atmosphere. They’re able to start picking up gamma rays.
The reason that we don’t get blasted with gamma rays and x-rays here on the surface of the Earth is we have different characteristics of our atmosphere predominantly the ozone when it comes to things like the ultraviolet that are absorbing different colors of light.
If you only have the amount of air that is between me and my computer screen, between me and the other side of the room, that amount of air isn’t enough to guarantee that all the x-rays and gamma rays are absorbed. If instead I have several miles of atmosphere, this several miles of atmosphere are able to make sure that pretty much none of these dangerous particles reach the surface of the Earth. It just takes a lot of distance.
Now at the same time, those molecules in the atmosphere don’t care about visible light. They happily let all the visible light pass through. Water in the atmosphere is another one of those things that likes to absorb. Happily, visible light passes right through all of the water vapor in the atmosphere.
At the same time a piece of paper goes nope, visible light is too thick to go through me, the gamma rays and x-rays are able to snake right between the atoms and molecules in the piece of paper.
Visible light can’t do that. It’s all about what absorbs what colors. What absorbs what wavelengths? Molecules in the atmosphere specifically absorb the gamma rays and the x-rays but they let through the visible light. A piece of paper the stuff in the paper is dense enough to block the longer wavelengths the bigger wavelengths of visible light but the x-rays and gamma rays pass right through.
Fraser: Hmm. It is counterintuitive. You think it’s so powerful but in many cases it just comes down to what absorbs what.
Pamela: Exactly and there are very specific fingerprints of colors which we talked about a couple episodes ago that are absorbed by different atoms and molecules.
We got lucky and live on a planet that’s safe for life because the specific contents of our atmosphere absorb these dangerous wavelengths.
Fraser: Like a radio will go through a piece of paper.
Pamela: Radio will happily go through a piece of paper.
Fraser: So will infrared.
Pamela: Infrared actually won’t go through that piece of paper.
Fraser: Oh that’s right, but radio for sure.
Pamela: Radio for sure. You can’t block your cell phone signal with a piece of paper.
Fraser: Right and so on both ends of the spectrum ha-ha it gets through. That is kind of neat. Okay, let’s move on.
Dawn Hoverson asked: “We’ve all heard of the tidal heating of Io and Europa but what I want to know is how much of the Earth’s internal heating is due to the lunar and solar tidal forces and how much is due to radioactivity and leftover heat from its formation?”
Io is a great example, right? Io is locked in a tidal battle with Jupiter. It’s getting squished and squeezed and this tidal heating makes it the most volcanic object in the solar system.
It would be cold and dead but because it’s getting smushed and squished the friction of this is keeping it hot and very volcanic. Is that going on with the Earth at all?
Pamela: You know I did a quick search trying to find heat generated with the Mares Plate Tectonics due to tidal forces and I just couldn’t find anything that talked about this as being anything significant.
What it turns out is the major reason that the Earth is actually warmer than it should be based strictly on how much light we receive from the sun. It actually turns out that things like radon, the radiation released by decaying particles in granite just all sorts of random decaying stuff scattered all throughout the Earth’s crust is the major source of heat here on the planet Earth. We do still have residual heat from when the planet was formed.
Fraser: Okay so if you took the Earth as a blob of molten rock, let it cool but kept shining the sun on it to warm it up a little bit and let it cool and let it cool we’re hotter than we should be after 4.6 billion years because of radioactive rocks inside the Earth.
Pamela: It’s all about the energy released by all those nuclear decays that cause radon in your basement. Or at least radon in my basement, I don’t know if you have radon in your basement.
Fraser: I don’t think we have radon in our basement. [Laughter]
Pamela: So yeah our planet is rather radioactive and granite is one of the major sources at least of day to day background radiation. We have all sorts of pockets of radioactive materials scattered all through our crust. All of those decays are releasing heat and keeping our planet nice and toasty warm.
Fraser: If we were moved into the orbit of Io then it would switch over to tidal heating would be the major force that is going on.
Pamela: But luckily we’re not there and so we have a much more stable planet and we’re happy for this.
Fraser: Yeah. I think we have run out of time. We will pick this up next week with more questions and more regular shows. Thanks a lot Pamela.
This transcript is not an exact match to the audio file. It has been edited for clarity. Transcription and editing by Cindy Leonard.