Podcast: Play in new window | Download
Subscribe: RSS
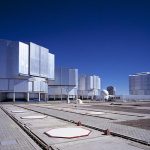
ESO Very Large Array. Image credit: ESO
When it comes to telescopes, bigger is better. But bigger is more expensive. Way more expensive. To keep the costs reasonable while improving the sensitivity of their instruments, astronomers use an amazing technique called interferometry. Instead of building a single huge telescope, you can merge the light from several telescopes to act like a much larger telescope. It’s a technique that has already revolutionized Earth-based observing – but just wait until it gets into space…
|
Shownotes
- Interferometry— JPL
- Episode #85: Detectors
- Observable Universe — Wise Geek
- Kuiper Belt Objects — Nine Planets
- Episode #41: Rise of the Super Telescopes
- Segmented mirrors — Wiki
- The Giant Magellan Telescope
- Waves and Particles –– Colorado University
- Constructive Interference — Windows to the Universe
- Collimated light
- The Very Large Telescope Interferometer
- Video: First Fringes of the VLTI
- PowerPoint presentation on Radio Interferometry by Jeffrey Kenney at Yale
- Terrestrial Planet Finder
- Atomic clocks — How Stuff Works
Transcript: Interferometry
Download the transcript
Fraser Cane: When it comes to telescopes bigger is better. But bigger is a lot more expensive, a lot more expensive. To keep the costs reasonable while improving the sensitivity of their instruments, astronomers use an amazing technique called interferometry. Instead of building a single huge telescope you can merge the light from several telescopes to act like a much larger telescope. It is a technique that has already revolutionized Earth-based observing. Just wait until it gets into space.
Okay Pamela, when I wrote that intro I kind of wanted to like not scare people. [Laughter] Interferometry, don’t let the title scare you. It is one of the coolest technologies that has been developed in kind of modern astronomy. I think it has led to part of this golden age that we’ve been talking about in astronomy.
As I said wait until we get to the space missions. Let’s talk about first the dilemma of building a gigantic telescope. What are kind of the limits of telescope technology right now?
Dr. Pamela Gay: You build giant telescopes for two basic reasons. One reason is you can get just so much more light. The more light you collect with your mirror, with your dish, with whatever light collecting surface you’re using, the fainter an object you can look at because you are getting more photons from that faint distant object or even that faint nearby object.
Cameras require a certain number of photons before they can go “yes I believe there is light here”. At the same time you also want to have high resolution images. You want to be able to make out the separation between close stars. You want to make out the details in galaxies. You want to be able to see all the bumps and wiggles in patterns of star formation regions’ blobs of gas and dust.
Both resolution and light collecting area depend in different ways on the radius of the telescope. For the light collecting area it is simply how much light are you gathering; it is what is the area of your detector. With resolution, all it cares about is how far apart the two edges of your detector are. How many wave lengths across is your detector? What’s cool is when you’re figuring up the resolution of the detector the detector doesn’t actually care if the middle part is there or not.
You can get the exact same resolution with a giant donut shaped mirror that is only maybe 4 inches wide in the donut part and 10 meters across from outer edge to outer edge as you’d get from having that entire solid mirror. That donut, amulus of mirror is going to weigh a lot less, is going to cost a lot less to produce and it doesn’t even care if it is a contiguous donut.
I could actually instead of having this ring of mirror, this ring of collecting surface; I could instead have maybe 4 different dishes at the north, south, east and west equivalence of that giant donut. As I break down to smaller and smaller areas, it gets cheaper and cheaper to build.
Fraser: Give me an example then of something where you want a lot of photons.
Pamela: A lot of photons are I’m trying to observe a galaxy at the very edge of the observable universe. This is a very faint object, very far away. We’re just not getting a lot of light. Here we want to collect more and more light or I’m trying to observe faint quiper belt object.
One of these blobs of frozen ice that is out around the orbit of Pluto, a very small very not necessarily reflective object I need to collect as much light as possible to try and see if it is there at all.
Fraser: Okay so it is like every photon is precious and if you can’t collect the photons you don’t even know that the thing exists.
Pamela: Exactly.
Fraser: What is the situation where high resolution is key?
Pamela: I’m looking at the star Betelgeuse. It is near enough by that if I use a high enough resolution detector I can actually make out the disc of the star. I can look at it and go, oh star spot.
I can look at it and I can measure how big it is. I know the distance to Betelgeuse and this allows me to actually calculate the physical size of the star.
Fraser: Then in this example Betelgeuse is giving off plenty of photons. No more photons are needed but the key is that you need to be able to have your resolution to be able to see the disc of the star, to be able to see sunspots.
As in the case as you mentioned before some kind of binary object where you’re trying to sense the separation between two stars. So then the traditional way is to build a big telescope. We talked this a bit in our rise of the super telescopes episode. Prices rise exponentially as the size of the telescope increases, right?
Pamela: Not only that but just the mechanical skill needed to get bigger than we are currently able to build, we’re just not there yet. Some of the largest telescopes in the world right now have 8 meter to 10 meter mirrors. These giant mirrors are right at our limit to spin cast them, to transport them to mount them so that gravity doesn’t deform them.
As we get to bigger and bigger mirrors we’re going to have to develop new technologies in using segmented mirrors; building new mount systems and being able to handle all of this weight without gravity deforming the systems. We’re reaching a point where engineering problems just as much as cost problems make it prohibitive to build these giant telescopes.
Fraser: There are plans in the works for 30 meter telescopes which will have all of these segments kind of lashed to make one great big telescope. The cost on that telescope like the Magellan is going to be enormous. You’re really kind of reaching the feasible limits but that is like a big light pocket right?
A big telescope like the Magellan is going to give you a 30 meter telescope to collect a whole lot of photons. That’s going to be seeing these faint quiper belt objects in these galaxies at the edge of the observable universe. Then here comes the solution, interferometry. So, what is interferometry?
Pamela: Interferometry basically goes light is particles that also act like waves. If you combine waves in a meaningful way, making sure that the peaks of one wave line up with the peaks of another wave they interfere in a way that we call constructive interference.
In this way you can collect a bunch of waves, line them up and it’s just as though you’ve collected all the waves at the exact same time. This sounds like a relatively simple idea. I go out, I collect my light, I somehow maybe using fiber optics, maybe using mirrors recombine this light and everything works.
Fraser: That’s kind of funny because it kind of sounds like gibberish to me. Let me just kind of parse this because I barely am wrapping my head around it.
You’re getting the light from one location and you’re getting the light from another location. You’re putting that light together? Is that right?
Pamela: Yeah.
Fraser: Then you are sort of seeing how the waves, as you said they construct or destruct one of those. I remember in physics we had the situation where you have waterways and you have two waves running into each other and if the two peaks come together then you get a double wave.
If the peak and the trough come together then you get flat water. I know light works the same way so I’m having trouble understanding how you can take light from two different positions and merge it together.
Pamela: This is where it gets very tricky. We’re really good as astronomers at doing this with radio waves. We know how to tune our receivers. We know how to detect the peaks and the troughs and the wave patterns very well.
With detectors like the Very Large Array, they know okay so the object is over in that part of the sky so I tilt all my dishes towards that object. I know exactly where on the surface of the Earth all of my telescopes are located very precisely.
I can use geometry to figure out this dish at this angle is this much closer to the object being observed; this other one is this much farther. They use different travel paths, different computers to take the signal from each of these telescopes and combine it with delays that allow the radio light that is received by each of these dishes in a slightly different location to be mixed. So it is as though the light was hitting each of the dishes that have left the object at the same time, hitting the telescope at the same time using artificial delays.
These artificial delays allow the peaks to stay lined up with the peaks and the valleys to stay lined up with the valleys and to get constructive interference and to artificially create a giant telescope aperture that gives you these extremely high resolution images.
Fraser: Are the two telescopes recording the same photon? You know, light could be a wave and you’re saying you’re trying to line up them together. Is that what’s going on that the photon is spreading out over a large area and so it is hitting the two telescopes and that is how you can kind of get at your better resolution? Is that what’s going on?
Pamela: We talk about light being what is called columate. This means the light that is coming off of an extremely distant star in an extremely distant galaxy, the light that is coming off of the source has the peaks and valleys within it lined up so that you get a coherent light beam.
So, they’re not detecting the exact same photon in two different dishes, but we’re detecting photons that are acting together in a columated fashion such that if I don’t have the moments at which I’m recording my data artificially lined up then I’m looking at a packet of photons that were released at one time, a packet that was released at another time. The peaks and valleys from these two different times might not be lined up.
I can get like you pointed out a trough lining up with a peak which gives me no light at all. It’s because it is coherent light coming off of the source that acts in what we call a columated fashion that we have all of these peaks and valleys, peaks and valleys lined up as the light travels through space.
Fraser: The timing is the key.
Pamela: Yeah, we have to maintain that lined up and we do it by shifting the signal from the telescopes until it is as though all the light was hitting the telescope at the same time.
Fraser: I guess I find that kind of, I’m sorry, I find that a little confusing as to why you say it is a columated light. I guess I find that part just a little confusing. A lot confusing which is why that is important?
I guess I understand if you don’t have the timing then it’s kind of like you have a telescope over here and a telescope over there. This one is taking images, that one is taking images that you could merge the images together – and this is what a lot of amateur astronomers do, right – they run video of some object that they’re trying to collect.
They take frame after frame and then they use image stacking software to kind of stack the images together to get a longer exposure but also to be able to remove the bad frames. That’s not what this is about.
Pamela: No.
Fraser: This is not taking two separate telescopes and merging the light together until you’ve got a thousand photons on the right telescope and a thousand photons on the left telescope. You put them together and you get a little bit better image. This is different. I think that is important.
Pamela: This is getting at increasing the resolution of the image.
Fraser: Right and as you said it is key to the fact that the photons were emitted at the same time and are connected is the way to put it?
Pamela: Connected is not quite the way to put it. First of all you do have the object varying. In theory I could take light from two different telescope dishes and combine it so that I have the peak of one wave combined with the peak of the next wave. In theory I’ll still get everything interfering in a way that allows me to get nice good sharp image.
But I’m observing the object in two different periods of time so I want to get a snapshot of the object in the now such that all of this light that I’m receiving traces the same behavior in the object.
There is also a matter of the resolution is directly related to how wide is my aperture. How wide is my reflecting surface? In this case that width is a reflection of how many wavelengths fits across the telescope.
If I have a ten meter optical telescope, it is going to have absolutely amazing resolution because optical light is extremely small. It is hundreds of nanometers. These are smaller than anything that you can believe or imagine because it is smaller than what you can see with your eye.
We have something that is several hundred nanometers peak to peak whereas with radio wavelengths I can have a ten meter dish and very, very poor resolution. An entire galaxy is nothing more than one pixel of well, this is light, this is dark. No you can’t see any details, all you know is there happens to be an object that emits radio over there somewhere.
This is because the radio wave ones are meters and meters in length. I’m trying to compare something that is hundreds of nanometers to something that is tens of meters in size. This is such a huge difference and it means a huge difference in the resolution.
Fraser: I think that is key though, that this is very tricky. With optical telescopes to line up the wavelengths between two optical telescopes you have to make sure that your wavelengths that are nanometers across are perfectly lined up.
That requires timing at an insanely complicated level. While you can imagine these radio wavelengths which can be meters across where you can kind of miss a little bit and it’s no big deal. To use this technique radio is the larger wavelength is where it really shines, right?
Pamela: Conveniently it is the radio wavelengths where we need this technology the most. A single dish has such terrible, terrible imaging resolution. It is only by starting to combine dishes that are actually spread across half the globe that we’re able to start getting really good resolutions.
One of the amazing things about this is with radio telescopes we’re able to very precisely record incoming radio light from distant objects in New Mexico, Massachusetts, and in Spain. Using dishes spread across the entire part of the planet that are capable of looking at some distant object at the same time we record the signal onto hard drives onto magnetic tapes. We record the timing of the observations.
Then artificially in a computer combine all of this light in a process that involves this neat thing called fringe finding where you carefully adjust the timing offsets between the data. We artificially combine everything to create this artificially large telescope.
With optical light we don’t have the ability to record the incoming light in the same way where we’re able to keep track of every peak and valley, every change in incoming photons. It is because of this difference where we end up resorting to things like using fiber optics where we physically delay the light travel time to the detector and physically combine the light so that it has the correct delays from one telescope to another.
Fraser: Let’s talk a bit about sort of what the set up of one of these inferometers looks like. There are a couple operating now and so sort of the visible light telescopes lay it out for us. What does this kind of look like?
Pamela: Visible light is still very, very experimental. There aren’t many systems in the world that are getting actively used for things that you and I would be able to see easily with our own light. The most famous of the systems is the Very Large Telescope Interferometer down in Chile.
We have the large 8.2 meter telescopes in the Atacama Desert that have the ability of using fiber optics, combine the light and get extremely high resolutions along what we call the baselines. These are the lines connecting one mirror’s center to another mirror’s center.
When you combine two telescopes you get extremely high resolution only along the direction in the image that has from one edge of one telescope to the other edge of the other telescope. Then you’ll get single dish resolution 90 degrees to that because we don’t have that added size to the telescope mirror in that direction of the telescopes aren’t spread out from one another.
Fraser: It’s like imagine a big long skinny telescope that is 8 meters high and how far apart are they?
Pamela: These are actually about 200 meters apart.
Fraser: Okay so it is 8 meters high and 200 meters wide. It is a very long skinny mirror. As you said it can go the other dimension too, right?
Pamela: Right so here they have multiple telescopes and they also have the additional little one meter telescopes that they can move around the facility and add in additional rays to end up getting as many as six different dishes on at the same time I believe.
By combining different mirrors in different ways all of this working in the optical – well here I have to say it is kind of a cheat to say the optical – it is the optical if you’re a snake.
This is near observing. There are systems that are working in what we do see as visible light but these are mostly systems that are still experimental. We’re still working on developing this technology.
Fraser: Right, the future has yet to be written.
Pamela: There are experiments in Sydney, in Hawaii, there are experiments all over the world trying to make this work invisible light but if you’re a snake, it is visible because we do have the strength you need for that.
Fraser: So, infrared and down sub millimeter into the radio microwaves, you’ve got the ability to merge these telescopes. As you mentioned the most amazing example of this is the fact that one whole half of the Earth can be called upon as one great big radio telescope. If what you need is a telescope the size of the planet there’s one available to you.
Pamela: Right and with the radio, we’re already doing this. We already have plans or at least different people have put together different plans, no one is working on building them at least right now that I know of to extend the baseline by putting dishes in orbit.
The plan to extend the baseline where instead of being confined to the diameter of the planet Earth, we’re instead confined to where in the solar system that we put our telescopes.
Fraser: We could put up one radio telescope on one side of the Earth’s orbit and another on the other side…
Pamela: Or stick one out on the moon. There are lots of different ways that we can start combining things.
Fraser: Right and the resolution goes up. Then there are plans in the works to develop space telescopes that would use interferometry. I guess our favorite cancelled space program that was where they were being planned, right with the terrestrial planet finder?
Pamela: Yes. This was the system that was looking to combine the light from three different telescopes to a central observing hub. It is a complicated system but in space it is using its lasers to very precisely locate the spacecraft.
We can get them spread out to much larger distances. Again this was a system that was looking to physically combine the light along these different path lights using physical delays.
We know how to do this. It’s not easy to do. It’s not cheap to do and this is why NASA hasn’t quite gotten around to actually doing it yet.
Fraser: Alright and I think the terrestrial planet finder this is one of those situations where the resolution is what you want. You want to be able to resolve a planet orbiting a star where it is a separation issue.
Obviously you want some photons but you really want to be able to just acknowledge its existence there. That’s not simple.
Pamela: Here we have to be able to resolve the planet separate from the star. We have to be able to block out the light from the star. It starts to become increasingly a more and more difficult system.
Fraser: When I first heard of the concept of interferometry I got really excited. I wondered if you could take, kind of like SETI at home. Amateur astronomers around the world could set up their telescopes, point it at some object that they’ve all been instructed to point it at and then gather light for whatever period of time.
They would then submit their images to some central clearinghouse that could then merge it all together to do interferometry in the visible spectrum. I emailed this to several scientists and I got some like polite laughter. [Laughter] Where was my thinking incorrect?
Pamela: Like I was saying earlier with the optical light the problem is we can’t just artificially recombine it later because you’ve been able to record with your CCD detector the over time variations and the life of peaks and troughs quite so conveniently.
With light you’re collecting photon after photon after photon and building an image with long integration times. You don’t do that in radio because the technology is just completely different. On top of that there are also all of the timing issues all of the positional issues.
This means that you can’t even do what you are suggesting if we replace all of the 12 inch amateur telescopes in people’s back yards with instead satellite dishes taken from the local cable television networks.
The issue with Inferometry is you have to know exactly the surface of the planet. You have to have atomic clock precision in the timing of your observations. Even with that, combining the data can be difficult to say the least.
The process of fringe binding the process of artificially lining up the radio data to get it to coherently combine, to get the peaks to line up with the peaks to enhance your signal is a complicated process. As you bring in the light from each different dish if you’re using for instance baseline interferometry you have to worry about things like the Russian’s telescope is off by three seconds. You have to worry about oh Spain was behind by half a second.
All of these small differences in timing have to be accounted for and they end up affecting your data as your telescopes move across the sky in several different ways and it is just hard to combine all of this data.
As you bring in more and more telescopes all of these small time issues become more and more things you have to worry about. It just becomes an untraceable puzzle fitting nightmare that no one wants to solve.
Fraser: It would work if we gave everybody an atomic clock and connected their telescopes by fiber optics and measured their position on the planet to an insane accuracy. Plus we would need to develop entirely new technologies and new kinds of computers.
Pamela: And we were able to wire the fiber optics in just the right way that we were able to exactly compensate for differences from multiple objects with multiple differences and travel time delays. It is just far too complicated of a problem.
Fraser: With radio dishes, it would be feasible.
Pamela: It’s all about the computer power there. Even then you would have to give everyone on the planet an atomic clock and military grade GPS.
Fraser: Oh well, all in the name of science. I’m up for it. [Laughter] Cool, well I think that this is going to be one of those technologies that over the next couple of years they will keep on developing the techniques.
They are going to crack it and I think that you’re going to really see some amazing research from ground-based telescopes and especially the space-based observatories that are hooked up in these baseline arrays. That’s going to really be amazing.
Interferometry – it is a complicated word but it is a very exciting technology. Read the stories when you hear something about some interferometer that comes online. It could be the next great technological advance. Thanks Pamela and we’ll talk to you at the next questions show.
Pamela: Sounds great Fraser, talk to you then.