Podcast: Play in new window | Download
Subscribe: RSS
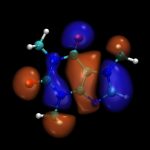
Caffeine atom with 3D electron orbitals. Image credit: Ivan S. Ufimtsev, Stanford University
We’ve talked about the biggest of the big, now let’s focus in on the smallest of the small. Let’s see what’s inside that basic building block of matter: the atom. You probably know the basics, but with ever more powerful particle accelerators, physicists are revealing particles within particles, announcing new discoveries all the time.
Ep. 164: Inside the Atom
|
Shownotes
- Ernest Rutherford — Nobel Prize page
- Plum Pudding model — Wiki
- J.J. Thomson, discovers the electron — Chemical Achievers
- How we saw the atom 100 years ago –American Institute of Physics
- Atomic theory; the early days — Vision Learning
- Atom Tutorial — Learn Chem
- The Structure of the Atom — Purdue
- Proton
- Neutron
- Electron
- Quantized energy — Kent Chemistry
- Pauli Exclusion Principle — GSU
- new spectroscopy technique to take “snapshots” of complex molecules — RSC
- Atomic mass is determined by the number of neutrons and protons that are present in the nucleus
- How Carbon-14 dating works — All About Archaeology
- Bohr Model — UTK
- Quarks — GSU
- More about quarks — Stanford
- Ep. #106: Search for the Theory of Everything
- Ep. #138: Quantum Mechanics
- Lambda particles — GSU
- What is the largest possible atom? Argonne National Lab
- 1995 press release about the discovery of the Top Quark — Fermilab
Transcript: Inside the Atom
Download the transcriptFraser: Astronomy Cast Episode 164 for Monday November 16, 2009, Inside the Atom. Welcome to Astronomy Cast, our weekly facts-based journey through the cosmos, where we help you understand not only what we know, but how we know what we know. My name is Fraser Cain, I’m the publisher of Universe Today, and with me is Dr. Pamela Gay, a professor at Southern Illinois University Edwardsville. Hey, Pamela.
Pamela: Hey, Fraser, how’s it going?
Fraser: It’s going very well. Very wet… but that’s the west coast in the winter.
Pamela: Yeah, we’re having the same thing here. Our wet is just a lot less wet than your wet.
Fraser: Yeah, oh, it’s crushing, it’s so wet… anyway, but, you know… it woud be nice to have a little bit of sun. I think my vitamin D level is low. So, we’ve talked about the biggest of the big, and now let’s focus in on the smallest of the small. Let’s see what’s inside that most basic building block of matter, the atom. Now, you probably know the basics, but with ever more powerful particle accelerators, physicists are revealing particles within particles, announcing new discoveries all the time. Alright, Pamela, so let’s come up with the simple, simple structure of the atom for starters and how we learned that. So, I think most of us know that atoms are the smallest particles that were first discovered that made up all of matter. We’re all made of atoms; molecules are made up of atoms. So what is the beginning structure of an atom?
Pamela: Well, initially we had it wrong. But sometimes it helps to start with what we did wrong to understand how we actually understand it today. So it all started with Rutherford, who came up with this idea for the model that said… ok, we have an atom; it’s made of a medley of electrons, of protons, of neutrons… we didn’t know all of those bits yet, but all this stuff is thrown in together. This was called the plum pudding model. J.J. Thompson, who was the discoverer of the electron in 1897, he decided he was going to test this plum pudding model. The idea was you take a very thin piece of gold sheet, and you fire electrons at it. If you get a plum pudding model, if you have this even smattering of everything put together, then you have all throughout it random scattering events. The electron flies in, it hits a proton, it flies off. It goes in, it hits a another electron, it flies off.
Fraser: Or it goes through and doesn’t hit anything.
Pamela: Or it goes through and doesn’t hit anything. But because you have this random mashing of everything together, the probability that it’s actually going to hit something is pretty high. It’s sort of like if you take 15 of your friends, and you tell them scatter yourselves about the driveway, and then you launch yourself on rollerskates down the driveway, you’re probably going to nail one of your friends. And they’re going to arrest you for crashing into the driveway. Now, the reality is, he started firing away at the gold foil, and most of the electrons went straight on through. And this was kind of confusing… because he had the wrong model. In reality, atoms have most of their mass—all of the neutrons, all of the protons—crammed down in the center. This is more of the case where you get your friends, you pile them in the driveway, and you tell them to get as close together as they possibly can… to stand in a really tight-knit huddle. Now in this case when you fire yourself down the driveway on your roller skates, you’re probably going to miss them and keep going and crash into whatever’s beyond your driveway. Well in this case when he fired, most of the stuff passed straight on through. But when he did occasionally make a solid connection, he got big scattering events and angles that indicated most of the mass was down in the center and most of the reactions were down in the center. It was a really neat experiment that forced him and everyone else to change how we looked at the atom.
Fraser: Right… as I recall it was an incredibly thin sheet of gold foil and you could imagine this constant beam of electrons firing out of this gun and them almost all going through, and then every now and then they were scattering back out, hitting detectors around the room.
Pamela: And it was Rutherford who interpreted this 1909 experiment to come up with our modern understanding of the atom. It’s just amazing to me to think that it was only 100 years ago, now that we’re recording this in 2009, it was only 100 years ago that we finally figured out what the model of the atom actually should look like.
Fraser: And so then we had the plum pudding model, so then that first model then was a tight bunch of protons and neutrons and then nothing…
Pamela: And then pretty much nothing. You have your electrons in a shell outside of this. It was actually the Bohr model a few years ago that started to really understand what the electrons were doing in their shell.
Fraser: But they first just thought that they were electrons going around the nucleus, kind of like a solar system, right?
Pamela: Right. And in fact, when you start looking at the equations that explain the electromagnetic force, that explain the pull between the electrons and protons, that force looks mathematically very, very similar to how gravity looks. And so it was easy to imagine that just as the planets orbit the sun, the electrons orbit the center of the atom… orbit the nucleus. Now the problem is, I can take a planet and throw it anywhere in the solar system, and as long as I throw it there with the right velocity, it’s happily going to orbit. But in atoms, it’s a little bit different. Instead we end up with these swarming clouds of electrons that are only allowed to orbit at said distances because the energies are quantized. Most of the atom is actually empty. One of the ways of looking at it that I adore is if you take a little green pea, and you toss it in the very center of a football field, the nearest electrons to that little pea in the center of the football field are going to be out at about the first row of seats in your standard stadium. That’s a whole lot of empty space.
Fraser: So the pea is the nucleus… those are the protons and the neutrons… and then those first electrons are the first row of seats.
Pamela: Exactly. Now it turns out that the electrons are sort of like people who hold tickets that are kind of open-ended. The electrons are limited to having certain energies, which means they have to orbit at certain types of distances away from the center of the nucleii. When we model this we use crazy shapes… what we’re actually explaining is clouds of stuff that exist at different distances. So, we have the inner-most orbits… these are the S1 orbits. Then we have a pair of orbits beyond that… each orbital level is restricted to having a very set number of electrons due to what’s called the Pauli Exclusion Principle, which we talked about in other shows.
Fraser: Right, but they’re like slots that can be filled and once they’re filled, then no more electrons are able to orbit in that shell.
Pamela: So, just as ticket holders fill up the rows of seats in a stadium, and your ticket says I’m restricted to a specific seat, the electrons are restricted to what slot… what energies they are allowed in the atom.
Fraser: And so if an electron gets out of one slot, then a different electron can go back into that slot, but the room has to be made first, right?
Pamela: Exactly. And it’s the specific slots that end up leading us to having the spectra of light that we see that lead to neon “Open” signs glowing in the particular shade of red they glow, and other neon signs in bars having the specific greens and yellows that we see.
Fraser: This is when electrons are going up and down levels, right?
Pamela: Exactly. And so here to continue our football analogy, you can almost imagine it takes energy to climb to the further energy levels because you have to trudge up the stairs, plant yourself in the seat higher up. Now for an electron to jump from one energy level to another, it has to absorb energy somehow… either through a collision or through the absorption of a photon… and it has to get just the right amount of energy so that when it’s done moving, it’s sitting in a new slot. It’s sitting in a new seat that already exists. It does it no good to get most of the way to the seat, because then it has to go back to where it started because it didn’t get all the way to the seat. And it doesn’t do it any good to get past the seat because now there’s no seat to sit in where you are now. So, the electrons have to be given just the right amount of energy to make the jump from one energy level to another, from one slot to another.
Fraser: So… sorry… so then what happens, right… If you have an electron that gets hit by a photon, it’s going to try to make the jump but it’s not enough energy to make it jump… what happens? Does it have new energy, or what happens?
Pamela: Well, you can end up just having a scattering event, where that photon comes in and it scatters off. You can have no interaction whatsoever… the photon just keeps going… this can go into collisional energy, it can go into kinetic energy. What’s amazing is atoms are a bundle of all sorts of different types of energies. It’s only when you add together the mass, add together the motion, add together what energy level within the atom everything is sitting in that you can get at the total energy of the system.
Fraser: Ok, so we’ve got this model, and I remember we drew them in chemistry class, right? You could draw the orbitals and that would help you figure out whether two atoms would combine together, right?
Pamela: Right. And, in fact, what you need in order to get a bonding… what you need in order to get two atoms to form a molecule is for there to be places that the electrons can be shared between the two atoms. Or, ways that the two atoms can fit together to fill electron energy levels together. So, in carbon, it has lots of empty slots just sitting there waiting for electrons to come in and fill the seats. Now, the neutral atom, the atom that doesn’t have any excess positive or negative electric charge, it’s going to sit there balanced out with these empty seats. Now, if another atom comes along, it can end up overlapping so that its filled seats line up on top of the empty seats of the other atom. This is a bit strange to think about. One way to think of it is if you take two egg cartons, it’s possible to fill one completely with eggs, and the other one half-way up with eggs, and then overlap the two cartons so that six eggs of one carton are filled, and then those little egg cardboard holders fill the empty holders on the other cardboard egg holder. Now you’ve bonded those egg boards together, and molecules can bond together in a very similar way.
Fraser: Right, and so one of the side-effects of sharing an orbital with an electron is that the two atoms will actually be bonded together, and this is what forms a molecule, right?
Pamela: Right. And so by sharing electrons, by holding everything together, we can get increasingly complex molecules. Building complex molecules is the easy part. What gets hard is when we try to build increasingly large atoms, instead.
Fraser: So now what really defines an atom with being a certain kind of element? Why is hydrogen hydrogen and gold gold?
Pamela: It’s all about the number of protons in the nucleus. If I have an atom and I strip every single electron out of the atom, I now have a very charged particle. But it’s still the same type of atom. I look in the core and I go… ah, you have 2 protons… you’re helium. Or, whatever the number of protons. What gets complicated is… ok, so gold can exist in many different forms depending on how many different neutrons it has in the center. We can get the same thing with carbon. In fact there are versions of carbon that are radioactive. What makes them radioactive is they’re not particularly stable because they have the wrong number of neutrons in the center. A nice happy carbon atom… it’s going to have six protons down in that core, and then it’s going to have another six neutrons. Now change those numbers and you start to get something that’s a whole lot less stable.
Fraser: Right, but if you add or remove protons, they become completely different elements.
Pamela: Yes.
Fraser: If you add or remove neutrons, you’re just changing how stable it is as an atom. And if you add or remove electrons, you just change its charge and how readily it’s going to bond to some other atom to create a molecule.
Pamela: So here we have Carbon 12 is nice, healthy and stable. Carbon 14 with just two extra neutrons… the numbers we use… that’s the sum of the protons and neutrons… Carbon 14… one atom… it will happily sit on a shelf for 5715 years, and then it just might decide it’s going to radiate away. It’s going to have that neutron decay… and in the process give off a bit of radiation. But more importantly, it’s the reason that we can use carbon dating. We can figure out when was something created and look at how much decay has happened and start to date archaeological sites all over the world.
Fraser: Now you mentioned the Bohr model as sort of the next more accurate understanding of the structure of the atom where the… where you’ve got these orbitals and it’s not like a little solar system. Is that still sort of the most accurate description? Because now we’ve got quantum mechanics to make things even more complicated.
Pamela: Well, Bohr was one of the people who started us down the quantum mechanics path. He was one of the ones who got us thinking… ok, energy is quantized… what does this mean? What are the implications? How does this relate to… well, we’ve got electrons going in circles, that means that there must be constant acceleration… what are the implications of this? And once we put all the pieces together, we had an entire new field of physics with which to torture undergraduates. And it’s that beginning model that Bohr created that has been fleshed out mathematically, but still remains the core of our modern model of how atoms work.
Fraser: Right. Now, let’s just draw this line in the sand, because the traditional understanding was proton, neutron, electron. That is the atom. But, scientists are never happy, and have been using particle accelerators to smash these things together to see what breaks… to see what comes out… So what was the next particle that was discovered inside the atom?
Pamela: The big kicker was we went from thinking first of all that atoms were as small as you could make things and then… no, no, no… totally wrong…. Now we have protons, neutrons, and electrons. We did all sorts of mean, awful, nasty things to electrons, and you really can’t harm an electron. Electrons are the smallest discrete piece of energy and mass and other characteristics that you can make that fit the electron description. You can’t break an electron into anything else… it can get absorbed… it can get turned into other things via processes, but the electron itself doesn’t have smaller things inside of it. Not so true for the proton and the neutron. This is where, as we started to smash and destroy and do evil, awful, nasty things to the protons and the neutrons… we realized that the protons and the neutrons were made of something different. In fact, they’re made of what we call quarks. This is a fairly new idea… it comes from the 1960s. It was proposed separately by a couple of different scientists… Murray Gell-Man and George Zweig… It was a way of trying to better understand the whole way of looking at particle physics in terms of… well, we have leptons… these are the electrons, the muons, the tau particles. We have bosons… these are the things that carry force, which are also fundamental and can’t be broken apart… So here we have the photon, the gluon, the weak force—which are the z and w bosons. Now how do you fit into this the proton and the neutron? Mathematically the way you fit into it is you come up with a family of six different quarks. Up and down are the ones that make up everything you and I deal with everyday. They’re the ones that make up protons and neutrons. But then there’s also charm and strange and top and bottom. It’s been a hunt that only ended in 1995 that we’ve been desperately trying to find in reality these particles that were proposed in the1960s.
Fraser: Now, I’ve got a million questions… let me see if I can put them into a kind of rational order… How many quarks are inside a proton or a neutron?
Pamela: Every proton and neutron is made up of three different quarks. So, if I have a hydrogen atom… just a straight old boring hydrogen with one proton and one electron. If I were to break it apart into as many possible pieces as I could break it apart, it would be made up of one down quark, two up quarks, and an electron… once I broke it all apart.
Fraser: Right, and now the down quark…. oh, and an electron?
Pamela: Right… because I’m breaking up the whole hydrogen atom.
Fraser: Right, right, right… of course. It’s got the electron already there. So now the up quark and the down quark doesn’t mean anything… it doesn’t mean it’s doing anything up or it’s doing anything down…
Pamela: They’re just names…
Fraser: They’re just names. How can they tell an up quark from a down quark?
Pamela: Well, when you add all of the pieces together, they end up carrying charge with them. And the different flavors… they actually have different masses. So the ups and the downs are the lightest weight of the quarks. The top quark… the one that was the most annoying to try to find.. this is the heaviest of the quarks. Now, a lot of times we tend to discuss the mass of things that are really, really tiny in terms of… well how much energy do I have to generate in order to get that particular amount of mass. The lightest weight is the up quark, and it takes 2.4 mega electron volts (MeV) to come up with an up quark. To get a top quark, that’s 171.2 giga electron volts (GeV). So there’s a huge difference between these two things.
Fraser: Right, and I think it’s important that people to listen to… we’ve done a bunch of shows about the search for the Theory of Everything and the search for the Higgs Boson… and trying to talk about particle colliders. That’s a whole show in itself… is how these particle colliders are using kinetic energy to freeze out mass through these collisions. I don’t think we want to go into that again in depth.
Pamela: Right.
Fraser: So, more energy… it takes more energy to create these heavier particles, with the top one being the heaviest and most difficult to find. So, really you crack open an atom… crack open a proton… three quarks are going to spill out?
Pamela: Three quarks are going to spill out.
Fraser: Always three. You’re going to weigh those three quarks, and that’s going to tell you what kind of quark they are. It’s almost like they’ll be quantized as well. They’ll always be one mass or a different mass or a different mass. And there’s six different… But they also come in groups, right? If you get one, you’re going to get the others, right?
Pamela: So you can build all sorts of different particle just by combining all the different types of quarks. We come up with tables of possible combinations… there’s six particles… you can combine them in all sorts of different ways. We know protons—up, up, down. We know neutrons—up, down, down. Then we start making stuff up. There’s lambda particles… these actually exist. This is a combination of an up, down, and strange. There’s sigma particles, which are two ups and a strange. And lots and lots of other combinations… But the one thing that holds always true is that the only ones that are stable are the ones made of ups and downs.
Fraser: Oh… so it’s kind of like molecules, right? You can mix and match your quarks and start making new particles… just whatever you can imagine. But, they just fall apart again in moments.
Pamela: Right. So we’re limited in what reality allows to exist. Reality periodically says ok… nice try… I appreciate the three quarks, but we’re done now, and it can say that in fragments of a second. The stable time for things like lambda particles are 2 x 10-10 of a second. You can’t even think about blinking that fast.
Fraser: So, then do any other of these particles exist in nature or are they only created in our labs?
Pamela: Well, some of the particles exist for passing moments in nature. We know that lambda and sigma and chi particles…. these regularly come out of different high energy events. Lots of times explosive, high power, collisional events… they create transitory things. They release huge amounts of energy and as that energy converts itself out to something else, it can pass through being… ah, I’m going to be this unstable particle… I’m going to be that unstable particle… before settling down to the combination of stable particles and light that fits what started best.
Fraser: Ok, then I guess my last big question is… have particle physicists been able to crack open quarks yet?
Pamela: As far as we know, it’s kind of like the electron… you can beat them all you want but they stay the same thing. The next big question for us is just how big can you make an atom? And this is where some of the particle accelerators have been doing really interesting work bombarding atoms with neutrons because the neutrons will eventually decay down into protons, and you can end up growing the center of an atom by bombarding something with huge numbers of neutrons and waiting to see what it decays into. There are mathematically various very, very large atoms that if you get just the right combination of protons and neutrons might be temporarily stable. We haven’t gotten there yet, but we’re working on it.
Fraser: Well, I know that we’re in the low hundred, right, in terms of the number of protons that you’re able to squish together. And for these Californium and Einsteinium and, you know, they last for just fractions of a second, and then they disappear again. I know the most heavy atom was created just a couple of years ago, now. And that record will continue to be broken. So you’re saying that they might hit some point where they’re stable again?
Pamela: Well, not so much stable, but they last slightly longer.
Fraser: Stabler…
Pamela: Right. So the highest atom that we’ve gotten to so far is, as near as I can tell, unpronounceable…. it’s Ununoctium. It’s abbreviation is Uuo. It’a a noble gas, and when you count up all of its protons and neutrons it has 294 crammed down in its center. So we’re getting to some hellaciously big atoms already, but there just might be some other ones up there that are waiting to be discovered mathematically.
Fraser: Alright, Pamela, I think that gives us a really good idea of the structure of the atom. It sounds so simple.
Pamela: It sounds so simple but it requires quarks and the strong force, which we have an entire show dedicated to… it requires neutrons to decay and electrons to jump levels. It’s a wonderful combination of all the different parts of physics.
Fraser: But what an accomplishment, I mean, over the last 100 years, essentially, they’ve gone from thinking atoms are little billiard balls to unraveling all the structure inside of it and really understanding how it all comes together. Some of the most basic research has been done in the last couple of decades. When was the top quark discovered?
Pamela: It was discovered in 1995. I remember it clearly because I wasn’t yet 21, and I was at Michigan State when the discovery was made. One of the senior faculty handed me champagne and said you’re going to drink. I had faculty-induced illegal drinking to celebrate the top quark.
Fraser: Yeah, and that’s only less than 15 years ago… so that’s amazing. Alright, Pamela, well thanks a lot, and we’ll talk to you next week.
Pamela: Ok, thank you very much. I’ll talk to you later.
This transcript is not an exact match to the audio file. It has been edited for clarity.